COF-999 is the latest stable covalent organic framework (COF) synthesized in the lab of Professor Omar M. Yaghi, University of California, Berkeley, USA. As reported earlier, COF-999 demonstrates exceptional CO₂ capture performance from open air, especially in humid conditions, while remaining chemically and thermally stable.
Here, Omar Yaghi talks to Vera Koester for ChemistryViews about the ability of this COF to adsorb CO₂ and its potential to solve the CO₂ problem, his research and the opening of the field of reticular chemistry, the use of chemistry to solve societal challenges such as climate change or water scarcity, how and why chemistry needs to be made more attractive and accessible, and the evolving role of the chemist.
Why COF-999? How do you decide on names or numbers for your molecules?
We choose the numbers roughly in the chronological order of discovery, and very often, I’ll ask the student involved, “What’s your favorite number?” Sometimes it’s their cat’s birthday, their own birthday, or simply a number they like if it fits in the order.
For a material with such a superior CO2 capture performance, we intended to give it a number that is easy to remember.
What inspired you to focus on CO₂ capture with COFs?
I’d like to first talk about CO₂ and MOFs: We’ve been working on CO₂ capture since we discovered metal-organic frameworks (MOFs) in the mid-1990s. We conducted some of the first CO₂ isotherms with MOFs—the very first isotherms involving CO₂ capture and its adsorption into the pores. In those early days, very quickly, once we were able to make the appropriate MOFs, we began exploring separations involving CO₂ and nitrogen, methane, and other gases involved in key industrial processes.
Once we made ultra-high surface area MOFs, we started looking at how much CO₂ could be stored in a single MOF. And it turned out, in one vessel of MOF you could store 18 times the amount of CO₂ compared to an empty vessel. This meant the CO₂ molecules are being compacted inside the pores much closer to each other than they would be without the MOF. The remarkable observation is that even though the MOF occupies volume in the vessel, it still holds 18 times more CO₂ than a vessel without MOF. All conditions being the same in terms of temperature and pressure. So that was exciting!
We soon shifted our focus to the challenge of CO₂ capture and have been working on capturing CO₂ from air and various gas sources for at least 17–18 years. The concept involves using amine solutions, which have traditionally been used to separate CO₂ from natural gas, largely made of methane. When you mine natural gas, depending on where you mine it from, it can contain up to 20 % CO₂.
Amines are great at capturing CO₂ because they are basic. CO₂ reacts with the amine to form carbamate or carbamic acid. Once CO₂ binds to the amine units, it needs to be released. For this, traditionally, one is required to heat water-based amine solutions, and water has a very high heat capacity. Think about boiling an egg. It takes a long time to heat the water. So, the problem is that this process consumes a lot of energy.
Also, when you start cycling, the amine solutions decompose and break down, creating an environmental problem of their own.
So, the idea was to find a way to attach amines to a solid material, as has been done with mesoporous silica, by either introducing amines into the pores or attaching the amine to the backbone. This approach aimed to replace heating a water solution with heating a solid, which has a much lower heat capacity than water, thereby saving energy. Still, the issue with cycling was that the silica materials were not sufficiently stable under basic conditions.
Over the past 20 years, our work on COFs has focused on progressively developing frameworks that are increasingly thermally and chemically robust. Once we had such COFs in hand we thought the time was right to attach polymeric amines covalently through strong bonds to the backbone, and it worked.
This means you can cycle. We performed 100 cycles without any decomposition—this is currently the highest number of cycles reported. It’s exciting! Based on this observed stability, it is estimated that the material could endure hundreds of thousands of cycles, enabling it to function effectively for many years.
And it works for taking CO₂ from the open air. We used Berkeley air, just outdoor air, to show that, in fact, it works under real-world conditions.
How did you test that?
We simply piped air from outside the lab into an instrument containing a column of the COF solid. Air enters one side of the column and exits from the other, where a mass spectrometer, GC, and IR are used to detect what’s coming in and out.
What did you detect?
We didn’t detect any CO₂ after the air passed through the column of COF. That’s a beautiful separation. When my student Zihui Zhou showed me the lab results, I was excited. I said to him, “I think that we have figured out a way of freeing the world from the extra CO₂ in the air.” It was a very memorable moment for both of us.
That’s the beauty of science, Vera, that for Zihui one discovery like this elevates him as a scientist from just an aspiring person into a recognized scholar. Now he had discovered a way to really have a big societal impact. Science is so amazing in transforming people’s lives on every level.
Figure 1. COF-999 in a vial, held against the backdrop of Berkeley’s iconic tower. (Image: © Omar Yaghi Group)
How does CO₂ behave in the pores of your materials and what role has water in this process?
With computations and experiments, we now understand what the CO₂ is doing in the pores, what is the equilibrium between carbamate, carbamic acid, and bicarbonate, all these CO₂-containing species that result from the adsorption of CO₂ into the pores, and we understand also the role of water in shifting this equilibrium towards the species that favor higher capacity for CO₂: the carbamic acid and the bicarbonate.
When water is involved, carbamic acid and carbamate are stabilized through hydrogen bonds and the formation of bicarbonates is promoted, which are also stabilized by hydrogen bonds with adjacent amine groups. In the absence of water, CO₂ reacts with primary or secondary amines, forming carbamic acid stabilized by hydrogen bonds with adjacent amines. This results in a 1:2 ratio of CO₂ to amine, which is less efficient because it requires more amines to capture the same amount of CO₂. In the presence of water, this ratio becomes 1:1 CO₂ to amine.
Computation was done with Professor Joachim Sauer from the Humboldt-Universität zu Berlin in Germany in collaboration with Professor Laura Gagliardi from the University of Chicago, IL, USA.
Because we understand what’s happening in the pores, we now have a strategy for potentially reaching double the uptake. We are looking into publishing details about this soon.
So what would that mean for bringing this into application and taking CO₂ out of the air?
At scale, we have about 1100 gigatons of CO₂ in the air, extra CO₂, the CO₂ that we pumped into the air because of burning fossil fuels. This CO₂ we need to take out of the air. We can stop burning fossil fuels tomorrow, but we still need to take it out of the air, because it affects the climate so severely.
Based on the results that we’ve published for COF-999, if you have a plant of 100,000 tons of this COF, it’s a large amount but it’s not too large for chemical companies, I mean they make millions of tons every year of stuff. A 100,000-ton plant would remove ½ gigaton of CO₂ out of the air within a year. It will take 2,000 years to take all CO₂ out of the air based on just that plant.
But if you put a plant in every city that has 2 million people or more in China, Europe, and the US, it will take 11 years to take the CO₂ out of the air. Now, if I put one of these plants in every city that has one million people or more around the world, it will take 3.5 years to rid ourselves of that extra CO₂. So, I’m just giving you a scale.
And now, when chemistry comes along and you double your uptake: Well, instead of 3.5 years, you can take it out in about less than two years. Noting that the captured CO₂ will be pumped under the ground for long-term storage. Currently, we are working on conversion of CO₂ to value added products. The goal is to close the cycle on capture and conversion to achieve carbon neutrality.
Figure 2. Schematic illustration of COF-999 with CO₂
This is amazing! So this could solve the climate crisis. However, it could also mean that we can continue with CO₂ pollution or even get worse?
It’s one way to think about it, but the scientific problem is solved. The rest is up to society to say, okay, let’s solve this problem, and then to prevent people from emitting, we put a cost on that, to discourage emitting and shifting our way of thinking.
How would the cost of using this COF compare to other CO₂ capture technologies?
I can’t say anything about the cost yet, because that always depends on who’s making it, what components, what improvements have been made. But let’s say it’s made from compounds that the industry is already working with, already making as bulk materials. The components of the COF are not exotic.
You reported using MOFs to harvest water from the air and are commercializing this. How do you foster broader thinking and skills that go beyond the immediate lab research and the success of fundamental research into successful applications? Or how has this developed for you?
I went into chemistry because I was excited about the beauty of molecules. When I was a young assistant professor, I wanted to build molecular objects that were beautiful. But I also wanted to address an intellectual challenge that was facing us as chemists. How do we make materials using the building block approach in an organized way? At that time, everyone said it couldn’t be done because when you start stitching things together with strong bonds, you face the crystallization problem, you end up making amorphous stuff.
We showed that that could be overcome by finding the right conditions to balance the kinetics and thermodynamics of crystallization.
I think, now we have an obligation to go beyond the basic science and to answer intellectual questions in our field. When we make discoveries, we are obligated to ask: What are they good for? How can we serve society that paid for that research, solving societal problems? I think the need for us to transform and solve societal problems is number one.
Number two, if I don’t take these inventions, I, my research group, people who make these discoveries, who would? Should we just stop there and hand it over to somebody else or should we strengthen our field and say, “Well, there’s no reason why a chemist couldn’t understand how to build a prototype and understand the mechanical engineering and the chemical engineering that goes into doing that.” I have much to gain and a motivation to make these following discoveries, too.
In so doing, you’re also transforming the way chemistry is done. No longer is a chemist “just” making molecules, or materials, but they’re also developing them in a way that could be placed in devices that actually society can use. There’s a lot of learning there. You’re amalgamating chemistry and engineering, mechanical engineering, chemical engineering, together. And now, there is AI to speed up the processes to explore a larger space: How do I predict what kind of materials I’m going to get? How do I predict what properties they might have? How do I optimize it? And then, how do I engineer it? All of that is connected computationally, using AI and robotics to help in making this cycle faster. I call this the AI discovery cycle. So, we should not be afraid to explore new frontiers.
What does all this mean for chemistry students?
I think we need to educate our students that there is no limit to their imagination and there is a demand from our students to transform chemistry, to make chemistry more relevant, to not only do the basics but tell us how I can contribute to a better world.
We need to make chemistry commensurate with the way and the speed with which society carries out things. Otherwise, chemistry would be left behind or shrink relative to other fields, because then big minds, who are ambitious and want to change the world, go into these expanding fields where there’s more opportunity and probably more rewards.
So, it is an essential to integrate AI into our workflows?
Chemistry will not be the most attractive topic if students go into the lab and are not using tools that they use elsewhere in their lives. On the contrary, it becomes exciting when they have tools that help them better understand concepts and make discoveries more effectively.
So will AI help further improve the accessibility of chemistry?
Yes. We are designing a platform, enabled by LLM, basic modeling, machine learning. This platform would allow more and more people to plug in and pursue research into MOFs and COFs for solving societal problems e.g., the water problem. That’s what we are aiming for by incorporating AI into our work. We’re trying to continue to lower the barrier so that chemistry becomes an easy field to get into and do productive things.
I think that with such platforms that enable people to plug in without having to have an instructor there 24 hours a day, you will see, first of all, the distribution of knowledge to be more evenly spread around the world. Why not have many scientists in many countries producing knowledge? I think they impact transformation for the betterment of humanity.
Maybe some scientists fear that competition will increase and their share of success will then be too small.
That was a very important decision that we made when we first discovered MOFs. One of my students said: “Professor, are you sure you want to report exactly how we made this amazing MOF because then everybody would jump in and start competing with us?” I said, “Absolutely.”
In fact, if you look at our papers, especially in the early days, we reported the preparation of those compounds in excruciating detail. And that was the best decision that we made. Yes, of course, it allowed everybody to easily plug into MOF chemistry. Indeed, I created my own ‘competition’ and that was the best thing I’ve ever done.
Colleagues around the world have carved out their own directions, becoming stars in their respective fields through their contributions. I believe this is better for everyone, rather than having success restricted to just a few labs. It scales the discovery. If more people can plug in, it means more ideas. This means more solutions are accessed. That’s very exciting.
That’s right, it’s exciting. Many of us have clichés in our heads not only about what successful research looks like, but also about what a successful chemist looks like—presumably still often an older, white man.
I think we need to heterogenize our activities. I mean, women, people from different geographies, different races, different backgrounds… I was just in South Africa and met many great chemists there. They should be invited to large chemistry meetings in Europe, in the US, and on the international stage because the quality of their science is very much to that level, but they don’t get that exposure, because in our minds we, unfortunately, think of scientists as you describe that the prototypical scientist is, looks like a specific person, but in fact, they come from all walks of life. What’s in their mind is all that you should be caring about and their contributions.
But what’s the point of talking about it, honestly? You’re not going to change that in society. The only way to change it is by continuing your scientific contributions and indeed the groundbreaking work.
What do you suggest to young scientists?
They need to understand that the path to success and the path to doing something that means something in the world is not easy, and you need to make sure that your work is not affected by your own thinking of who looks like a successful scientist, you need to forge ahead.
I think that’s what young people need to keep in mind, that at the end of the day changing society is going to be a lot slower than we like. It’s a lot faster to show that in fact, you can contribute just as well as everybody else and maybe even more. I think that kept me going. That kept me excited, and I think it would have been discouraging had I learned what society’s disposition is, very early on in my career.
But, you know, it’s complex because for some of us, like me, I’m an immigrant from a refugee family, you always have in your mind that failure is not an option. Going back is not an option. Going forward is the only way, and so this is a singular thing.
Move forward and deal with whatever your problem is right now; nobody’s going to hand you anything on a platter. Maybe I had to work a little harder than everyone else to be recognized, but that hasn’t been all that bad for me.
Thank you very much for sharing these insights and we are looking forward to hearing more about COF-999.
Omar M. Yaghi studied chemistry at the State University of New York-Albany, NY, USA, and the University of Illinois-Urbana, USA, where he received his Ph.D. in 1990. He was a National Science Foundation (NSF) Postdoctoral Fellow at Harvard University, Cambridge, MA, USA, from 1990 to 1992. Omar Yaghi joined the Arizona State University, USA, as an Assistant Professor, and in 1999, he moved to the University of Michigan, USA, as Robert W. Parry Professor. From 2006 to 2011, he served as Irving and Jean Stone Chair in Physical Sciences and Christopher S. Foote Professor of Chemistry at the University of California, Los Angeles, USA.
Since 2012, Omar Yaghi has been the James and Neeltje Tretter Chair Professor of Chemistry at the University of California (UC), Berkeley, and an Affiliated Scientist at the Lawrence Berkeley National Laboratory, CA, USA.
He is the Founding Director of the Berkeley Global Science Institute, which builds research centers in developing countries and supports young scientists. He is also the Co-Director of the Kavli Energy NanoSciences Institute (Kavli ENSI), focusing on the basic science of energy transformation on the molecular level, the California Research Alliance by BASF (CARA) supporting joint academia-industry innovations, as well as the Bakar Institute of Digital Materials for the Planet (BIDMaP) which aims to develop cost-efficient, deployable metal-organic (MOFs) and covalent organic frameworks (COFs) to address climate change impacts.
Omar Yaghi has received numerous prestigious awards, including the Wolf Prize in 2018, the Royal Swedish Academy of Sciences Gregori Aminoff Prize in 2019, and the Solvay Prize, Balzan Prize, and Tang Prize in 2024. Omar Yaghi is an Elected Member of the U.S. National Academy of Sciences as well as the German National Academy of Sciences, Leopoldina, and serves on numerous Editorial and Advisory Boards of numerous scientific journals.
Selected Publications
- Carbon dioxide capture from open air using covalent organic frameworks,
Zihui Zhou, Tianqiong Ma, Heyang Zhang, Saumil Chheda, Haozhe Li, Kaiyu Wang, Sebastian Ehrling, Raynald Giovine, Chuanshuai Li, Ali H. Alawadhi, Marwan M. Abduljawad, Majed O. Alawad, Laura Gagliardi, Joachim Sauer, Omar M. Yaghi,
Nature 2024.
https://doi.org/10.1038/s41586-024-08080-x - Docking of CuI and AgI in Metal–Organic Frameworks for Adsorption and Separation of Xenon,
H. Wang, Z. Shi, J. Yang, T. Sun, B. Rungtaweevoranit, H. Lyu, Y.-B. Zhang, O. M. Yaghi,
Angew. Chem. Int. Ed. 2021, 60, 3417–3421.
https://doi.org/10.1002/anie.202015262 - ‘Eye’ of the molecule—a viewpoint,
S. Lee, O. M. Yaghi,
Faraday Discuss. 2021.
https://doi.org/10.1039/d1fd00032b - The Reticular Chemist,
O. M. Yaghi,
Nano Lett. 2020, 20, 8432–8434.
https://doi.org/10.1021/acs.nanolett.0c04327 - Practical water production from desert air,
Farhad Fathieh, Markus J. Kalmutzki, Eugene A. Kapustin, Peter J. Waller, Jingjing Yang, Omar M. Yaghi,
Science Advances 2018, 4(6).
https://doi.org/10.1126/sciadv.aat3198 - Weaving of organic threads into a crystalline covalent organic framework,
Y. Liu, Y. Ma, Y. Zhao, X. Sun, F. Gandara, H. Furukawa, Z. Liu, H. Zhu, C. Zhu, K. Suenaga, P. Oleynikov, A. S. Alshammari, X. Zhang, O. Terasaki, O. M. Yaghi,
Science 2016, 351, 365–369.
https://doi.org/10.1126/science.aad4011 - The Chemistry and Applications of Metal-Organic Frameworks,
H. Furukawa, K. E. Cordova, M. O’Keeffe, O. M. Yaghi,
Science 2013, 341, 1230444.
https://doi.org/10.1126/science.1230444 - Reticular synthesis and the design of new materials,
O. M. Yaghi, M. O’Keeffe, N. W. Ockwig, H. K. Chae, M. Eddaoudi, J. Kim,
Nature 2003, 423, 705–714.
https://doi.org/10.1038/nature01650 - Systematic Design of Pore Size and Functionality in Isoreticular MOFs and Their Application in Methane Storage,
M. Eddaoudi,
Science 2002, 295, 469–472.
https://doi.org/10.1126/science.1067208
Also of Interest
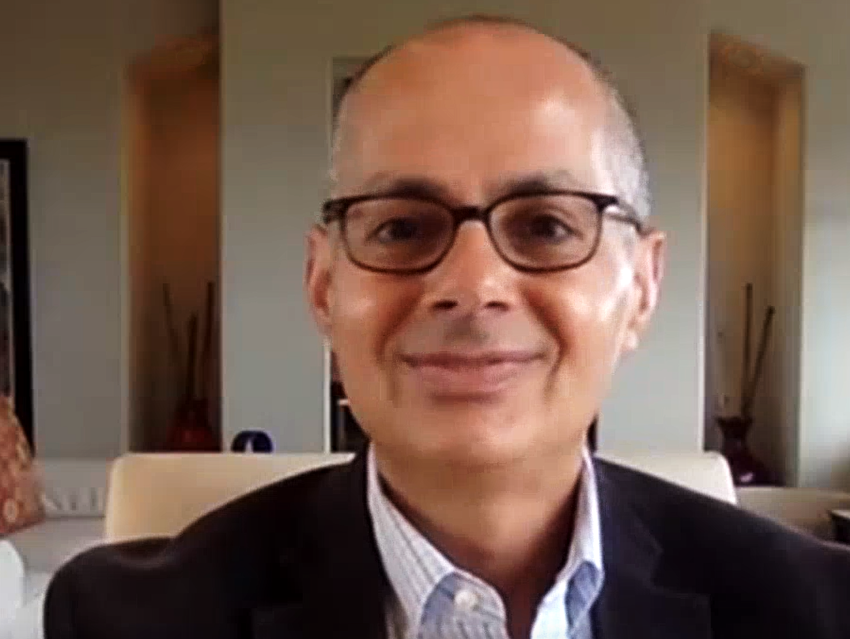
Omar M. Yaghi about his research, the discovery of MOFs, supporting young academics, and our responsibility as scientists—Laureate Interview for the August Wilhelm von Hofmann Medal of the German Chemical Society (GDCh)—ChemistryViews in cooperation with GDCh
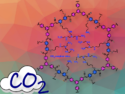
COF-999, a stable covalent organic framework, demonstrates exceptional CO2 capture performance from open air, especially in humid conditions
-
Covalent Organic Frameworks with Ester Links,
ChemistryViews 2020.
First crystalline ester-linked COFs related to common polyesters - Stable Olefin-Linked Covalent Organic Framework,
ChemistryViews 2019.
First unsubstituted olefin-linked COF was synthesized via aldol condensation
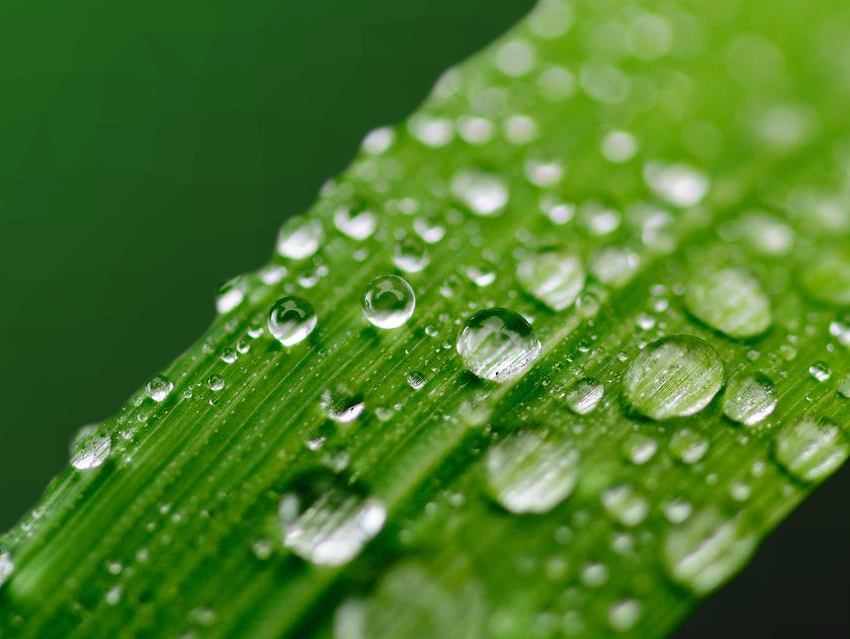
Spotlight: Capturing Water from the Atmosphere
Can we harvest drinking water directly from the air around us? – Examples from nature and research